Iron taken to the extreme
Categories : Recherche Résultats
paru le 11-10-2021 (14:43) - Updated on 10-29-2024 (16:57)
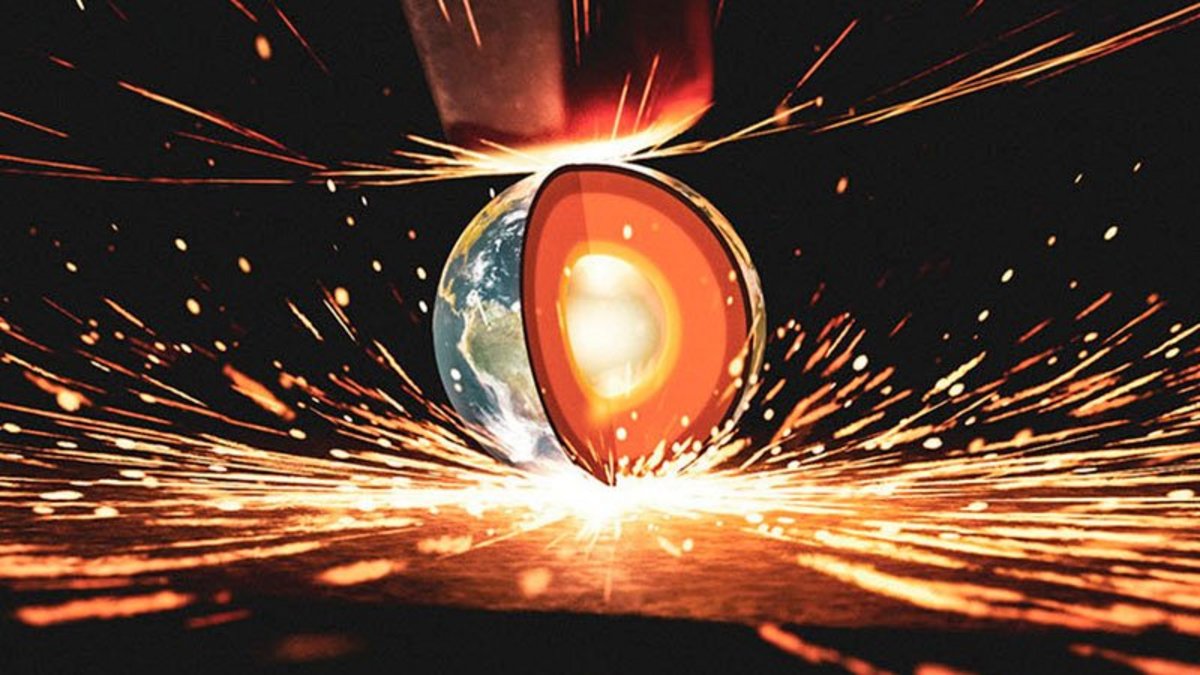
A car body that gets crushed in an accident: knowing what happens to metal at the atomic level − how it deforms while retaining its metallic nature − is almost routine for materials specialists. But what happens when the shock is much more violent, as for example when a space probe returns to Earth and compresses the air in front of it to such an extent that temperatures at the front of its fuselage reach thousands of degrees? Or worse, when the metallic cores of two planets collide?
Of course, it’s impossible to take measurements in situ. To find out what’s going on, we usually have to set in motion costly simulations that sometimes last several years. ‘These simulations are costly and complex because they require a wide range of skills, since the calculations involve different scales and therefore different fields of physics’,explains Sébastien Merkel. ‘Few teams, apart from the big national laboratories, master this entirechain.’
A unique experience
This is why the publication of research coordinated by Sébastien Merkel of the Materials and Transformations Unit (Umet¹) in the leading physics journal, Physical Review Letters,is a first. No longer a simulation, but a real experiment: the team succeeded in reproducing and observing in the laboratory, for just a moment, what happens to iron under such extreme conditions. The window of time is tiny, but oh so precious: ten billionths of a second, a fleeting flash that nonetheless takes us into an unknown world. A world where iron reaches an unprecedented state of extreme solidity.
Like many solid-state materials, iron is a crystal, i.e. it’s made up of a regular, geometric arrangement of atoms. At the usual temperatures and pressures on the Earth’s surface, this arrangement is made up of cubes aligned in the three spatial dimensions, with iron atoms forming the centre and vertices. Under the extreme conditions of the experiment, however, there isn’t enough space: the iron atoms arrange themselves in hexagonal prisms. This was predicted and well observed by experience. What was less clear, however, was how the iron would react to the deformation moving through the sample.
The team’s results showed that a crystal tilting phenomenon was occurring, a phenomenon that is well known to mineralogists because its consequences can sometimes be observed with the naked eye, resulting in the formation of astonishing interlocking crystals calledmacles (‘twinning’). In the case of iron, the very high stresses it undergoes lead it to adopt a new configuration: the hexagonal prisms rotate 90 degrees in relation to each other and interpenetrate, forming a very solid material. The very important contribution of the team is precisely to have been able to measure the conditions and time required for the activation of this mechanism − a tiny billionth of a second.